How is it mesured ?
This page presents the most commonly used techniques in environmental monitoring, particularly by contributors to the RNM.
Sampling and analysis plans
Regulatory environmental monitoring of basic nuclear installations is adapted to each type of installation (nuclear power plant, factory, laboratory). Analyses performed on each sample follow predefined plans developed based on emissions (type of emission, radionuclides and activities emitted) and its event history (incidents and past emissions).
Sample preparation
Upon receipt at the laboratory, samples are processed and conditioned according to the radiological analyses they will undergo. The analysis of radioactivity in a sample taken from the environment begins with its preparation.
This ranges from simple conditioning in a standardized container to more elaborate preparation such as drying, calcination, or lyophilization.
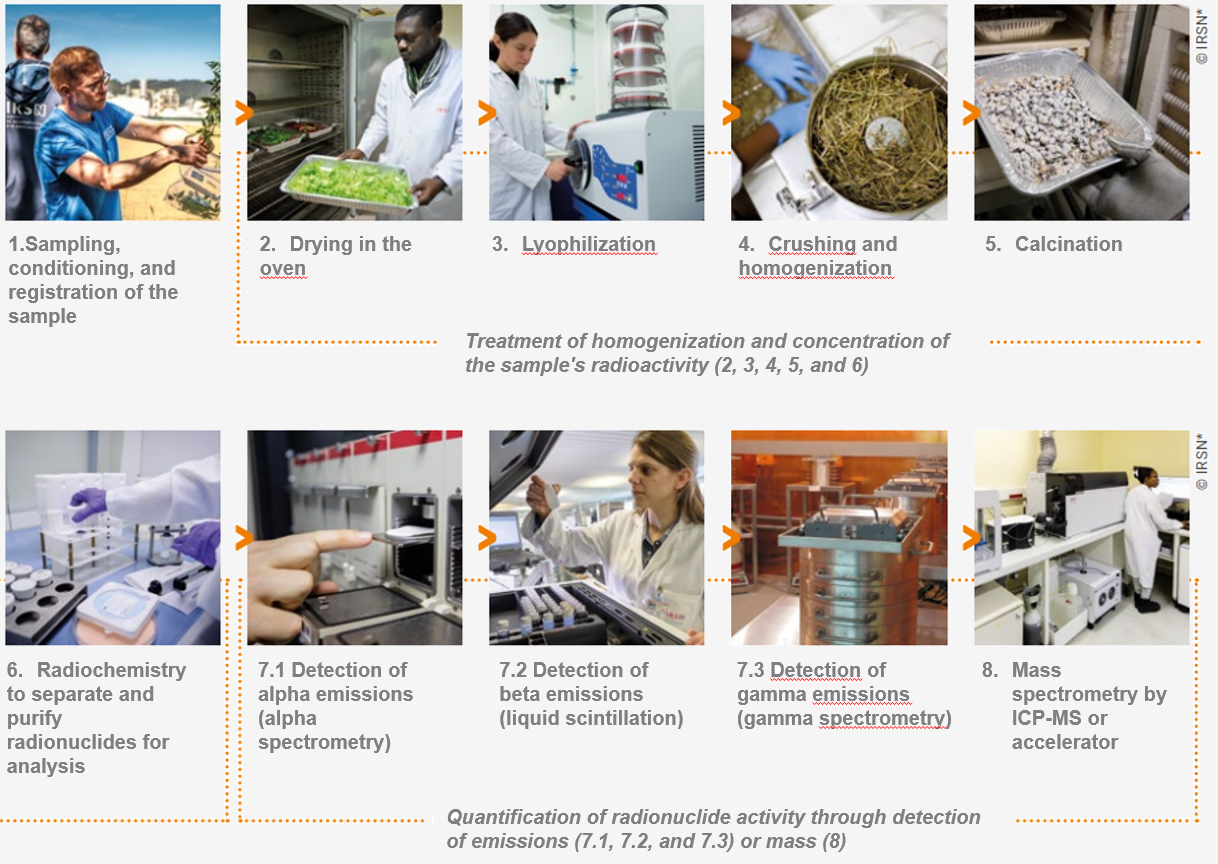
Generally, the various sample processing techniques aim to concentrate as much radioactivity as possible in a minimum volume to detect the presence of radionuclides at low, or very low levels. In some cases, sample concentration begins at the time of sampling using specific devices (examples: aerosol sampling, suspended matter sampling...).
In most cases, the analysis does not require the use of the entire sample. It is then homogenized beforehand so that the test sample does not bias the representativeness of the measurement. Most monitoring actors also archive part of the analyzed samples (filters, dried soils and sediments, dried or calcined fauna and flora samples) for possible future analysis using complementary or more advanced methods.
The need to concentrate the sample or extract the radionuclides to be measured involves the implementation of a laboratory that can perform, at the highest quality level, all or part of the steps leading to the characterization of radionuclides present in a sample.
Radiological analysis methods
Numerous methods for measuring radioactivity in the environment are used depending on the radionuclides sought, the matrices to be analyzed, and the metrological performance aimed for. They can be generically divided into two main types: measuring the radiation emitted by radionuclides, alpha, beta, or gamma, and measuring the mass abundance of radionuclides present to determine their activity (this is called mass spectrometry). While the first type was historically the first and remains the most widespread, mass spectrometry analyses are increasingly used today, offering incomparable performance for radionuclides sometimes complex to measure by other techniques, such as carbon-14. This section thus presents a synthetic overview of the main techniques used today in environmental sample measurement laboratories.
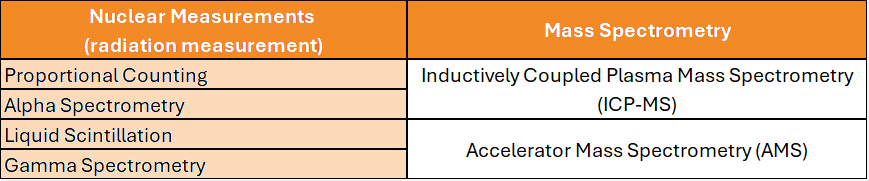
Proportional counting
Proportional counting is notably used for measuring global alpha and beta activity indices in environmental samples, particularly water. It can also be used for measuring beta emitters such as strontium-90 after a radio-chemical phase. Preparation is necessary before measurement. This preparation aims to obtain a thin deposit of material to be analyzed on a cup, which is then placed in the counter.
This thin deposit results either from the evaporation of a solution or from the ash deposit of the sample's incineration (e.g., aerosol filter).
The detector uses gas ionization to measure α and β radiation: particles emitted by the sample will strip electrons from atoms (ionization) of a gas present in a chamber, thus generating charged ions, and therefore a measurable electric current proportional to the radiation.
Gamma spectrometry
Gamma spectrometry is a measurement method that identifies and quantifies gamma-emitting radionuclides present in a sample, based on the detection of X and gamma photons emitted during their decay. It plays an important role in environmental monitoring, given that many natural or man-made radionuclides emit this type of radiation.
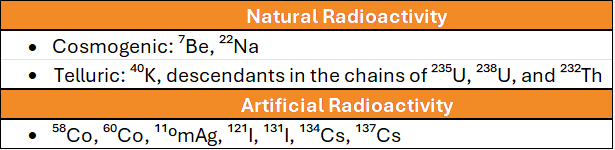
The measurement can be performed with different types of detectors, the most used being semiconductor detectors (e.g., high-purity germanium, GeHP) and scintillation detectors (e.g., thallium-doped sodium iodide, NaI(Tl)). It is a fast, non-destructive technique that allows measuring all gamma-emitting radionuclides present in the sample at once. However, the analysis can be complex depending on the type of sample, the number of radionuclides present and their characteristics, and the activity levels encountered.
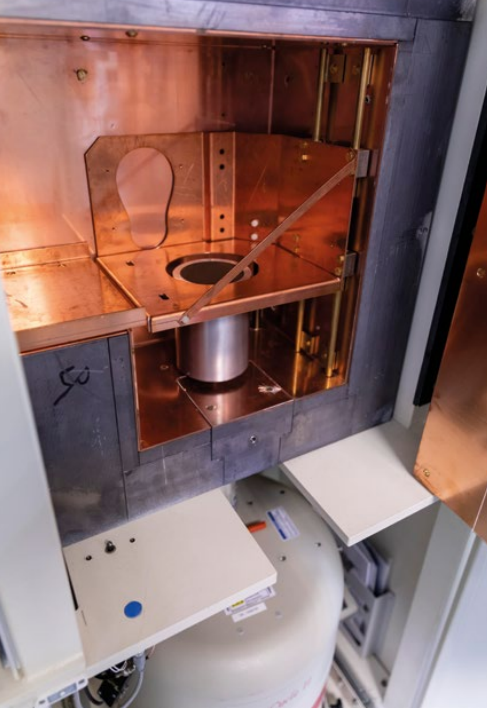
Samples can be analyzed in liquid or solid state and are conditioned in containers of various shapes ("geometries"), adapted to the quantities to be analyzed.
Depending on the radionuclides and expected activity levels, the sample may be pre-treated (drying, calcination, concentration). For example, to detect radionuclides with very low activities, the sample will be calcined to concentrate it as much as possible in a minimum volume. Gamma photons emitted by the treated sample interact with the detector material, depositing all or part of their energy. These interactions produce electrical pulses proportional to the deposited energies, which are collected and processed by an electronic system to be converted into numerical values. The classification of interactions in a histogram giving the number of detected events based on the deposited energy allows obtaining a spectrum ranging from a few keV to several MeV.
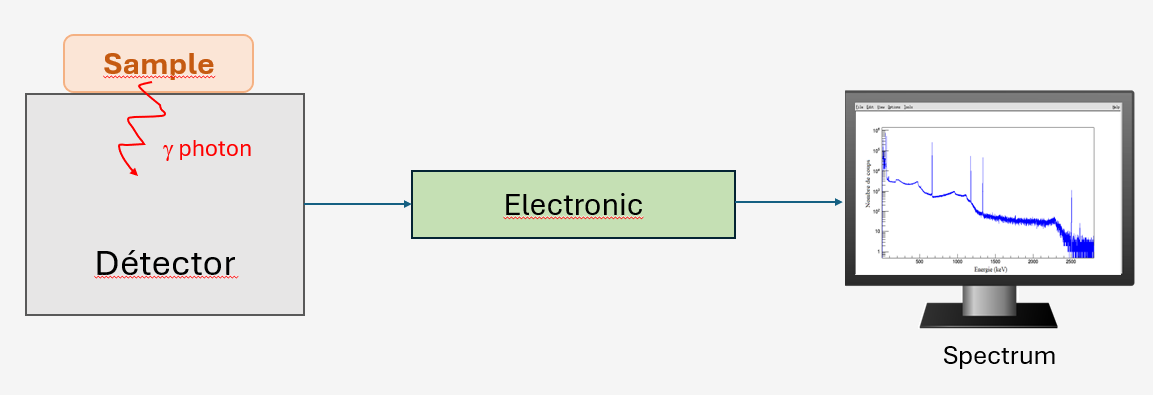
The spectrum is characterized by a continuous background and the presence of several peaks:
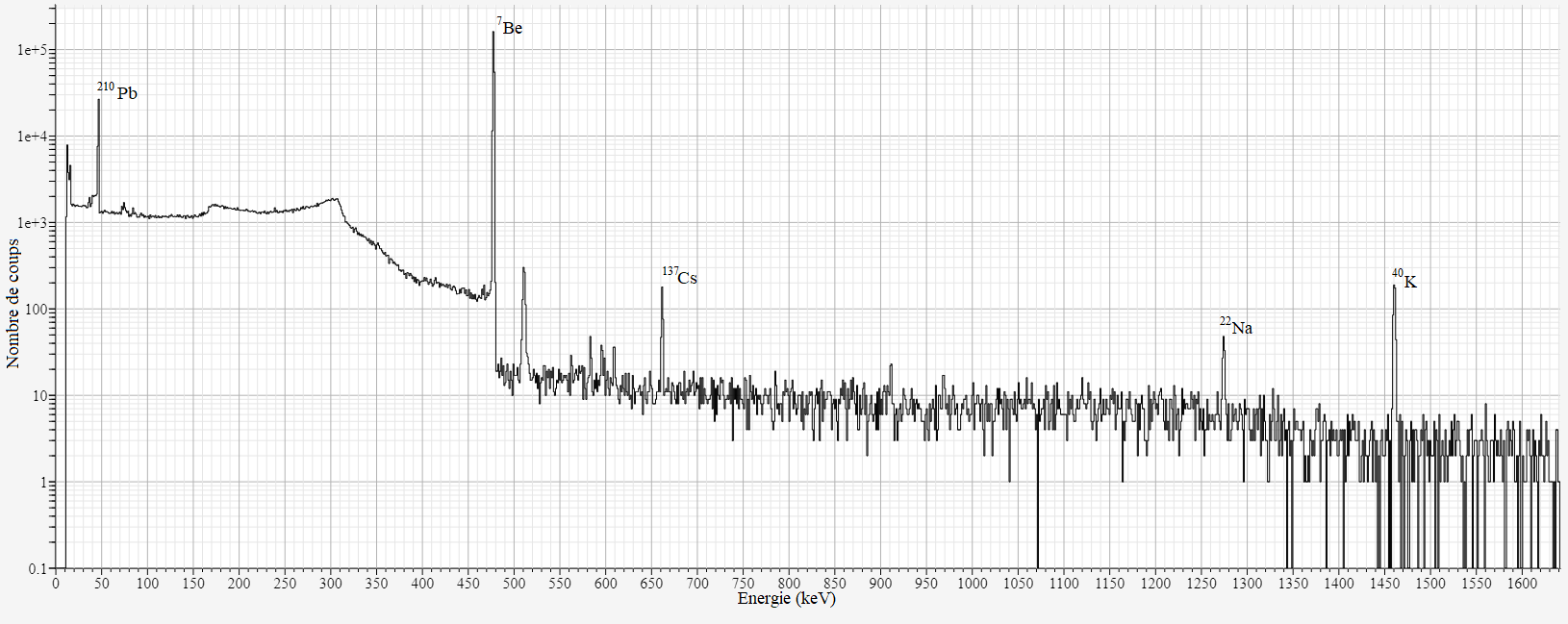
The number of detected events in a peak allows determining the activity of the associated radionuclide. Indeed, the activity depends on the number of counts in the peak, the emission intensity of the detected photon, the detection efficiency of the measurement system, the counting time, and various correction factors.
Activity levels in environmental samples can be low (up to a few mBq per sample), requiring precautions to protect the detector from ambient gamma radiation (radioactivity of materials surrounding the detector, cosmic radiation). To do this, a lead shield surrounds the detection system to reduce external background noise (natural telluric radioactivity, measured sample nearby), and the detector elements can also be selected for their low content of radioactive impurities ("low noise detector"). The component due to the presence of radon in the air can also be reduced by air renewal in the measurement room or by sweeping the detection chamber with a nitrogen flow. To further reduce background noise, the contribution from cosmic radiation can be decreased by adding an anti-cosmic system or by installing the detector in an underground laboratory. Counting time can vary between a few hours and several days depending on the sample to be analyzed, the goal being to have a sufficient number of counts in the studied peaks.
Alpha spectrometry
Given the low penetration of alpha particles, this radiation does not pass through matter. This low penetration requires removing all matter from the environmental sample except for the chemical element to be measured. This purification operation results from a thorough chemical treatment, summarized below.
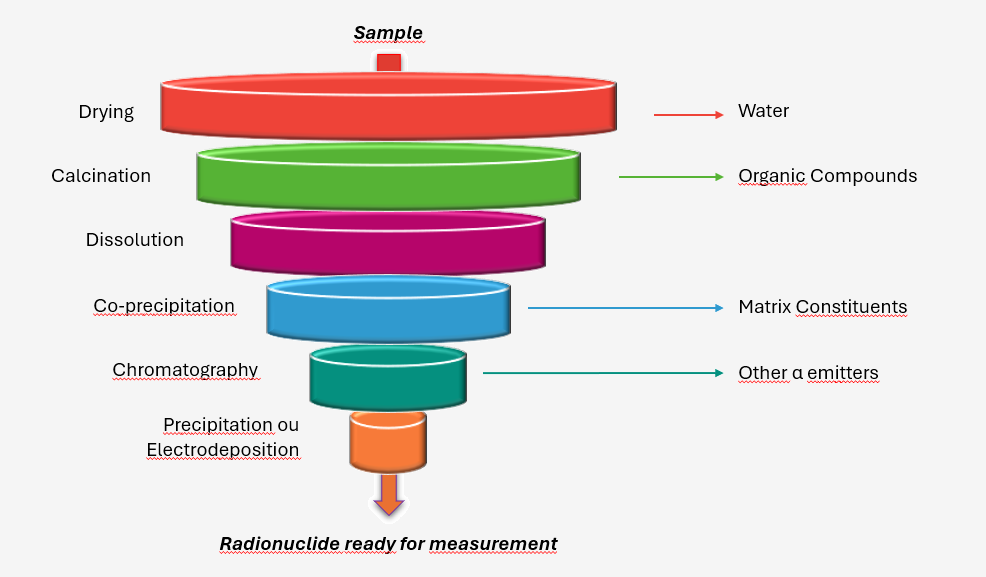
First, solid samples are dried and calcined to respectively eliminate water and organic compounds contained in the sample. A solution is then prepared hot with concentrated acids (HNO3, HCl, HClO4, HF). Separation steps isolate it from the rest of the matrix, and thus from other alpha emitters to avoid interferences (two radionuclides can emit alpha rays of the same energy). The duration of the radio-chemical treatment can reach 3 weeks depending on the radionuclide, the type, and the mass of the sample to be analyzed.
Samples resulting from radiochemistry (in the form of stainless-steel discs or filters) are placed in a counting chamber equipped with a silicon-based semiconductor detector. Alpha particles emitted by the sample interact with the detector placed almost in contact with the sample, creating an electric current in it, whose amplitude and intensity are proportional to the activity and energy of the alpha particles. The generated signal is converted into an exploitable energy spectrum by the measurer. Acquisition durations are generally 2 to 3 days to reach decision thresholds compatible with monitoring needs.
Liquid scintillation
Liquid scintillation is the preferred method for measuring beta-emitting radionuclides such as tritium, carbon-14, or strontium-90. For this measurement, the radioactive solution is mixed with a scintillating liquid that transforms the decay energy into luminous radiation.
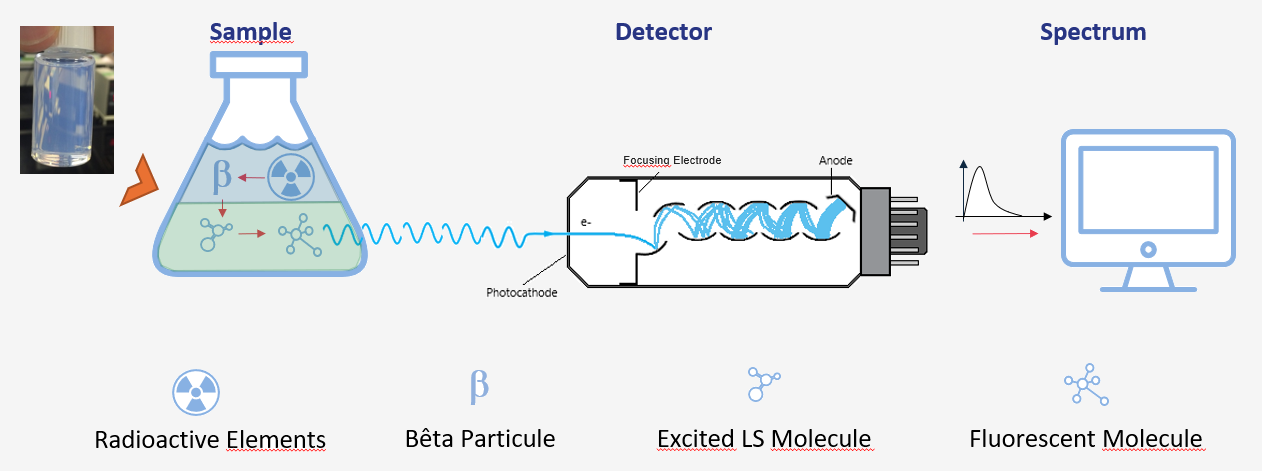
This technique, widely used in measurement laboratories, is reliable and relatively easy to implement. Its main advantages are detection efficiency and the absence of a physical barrier between the radioactive solution and the scintillator (both being combined in a homogeneous solution). This configuration allows detecting low-energy radiation with low detection limits, particularly relevant for environmental activities. However, for complex samples like those from the environment, this technique often requires preparation and chemical separation to avoid interferences during measurement.
After chemical treatment, the sample in solution is mixed with scintillating liquid. The latter is mainly composed of an organic solvent and scintillators (scintillating molecules) whose role is to transform beta radiation into visible radiation. The solvent is excited by beta radiation, and the scintillators emit light when the solvent is excited. Photomultipliers then amplify the light emitted by scintillation. Most recent counters include automatic sample feeders to measure series of samples and thus increase laboratory capacity.
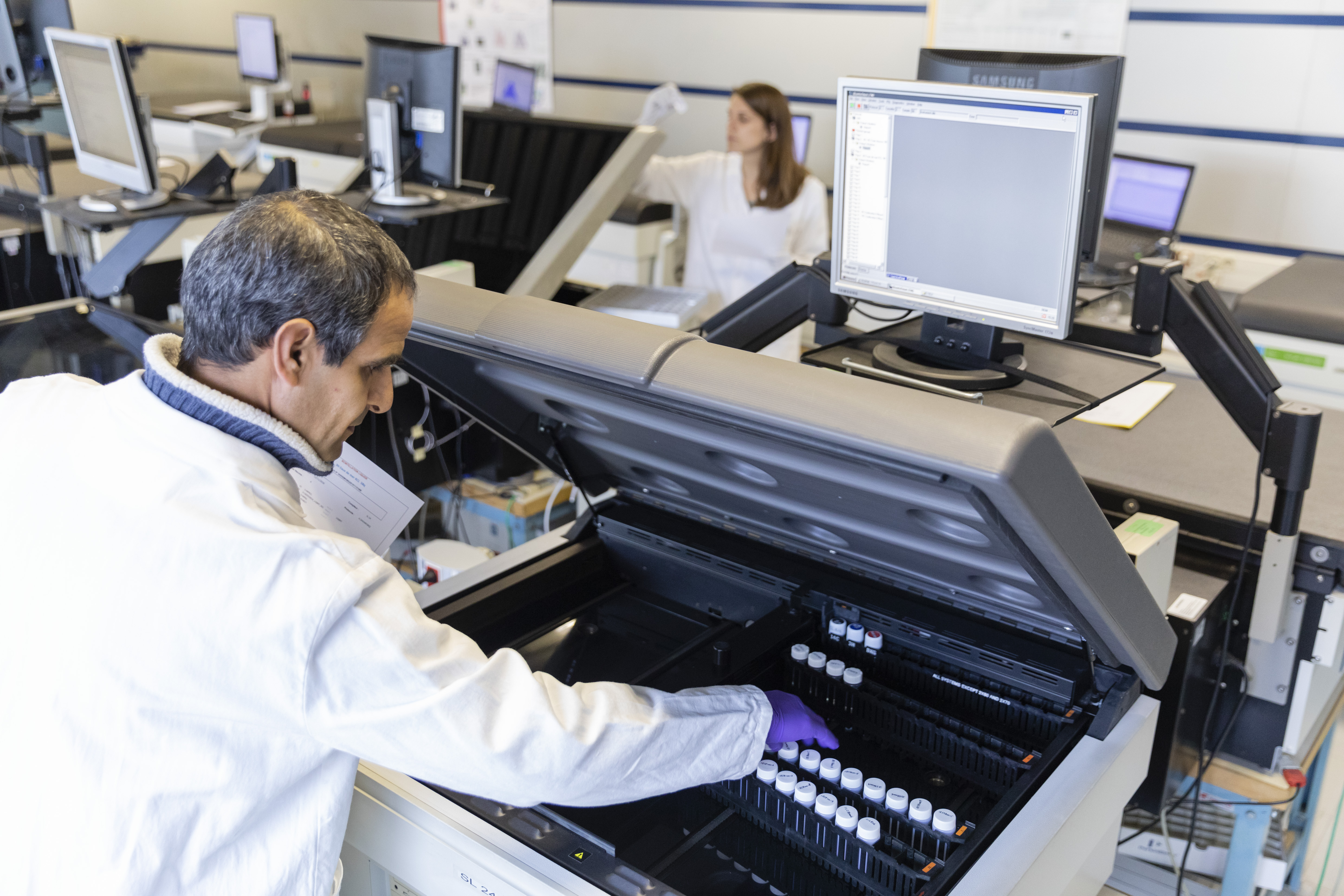
The obtained signal is processed by an analyzer and presented as an energy spectrum. As with alpha spectrometry (see above), the surface of the energy peaks in the spectrum provides the activity of the radionuclide contained in the initial sample.
Inductively Coupled Plasma Mass Spectrometry (ICP-MS)
Inductively Coupled Plasma Mass Spectrometry (ICP-MS) is one of the most sensitive analytical techniques for identifying and measuring a wide range of elements present in environmental samples. These can be particularly complex and difficult to process, especially due to their highly variable chemical compositions, requiring a radio-chemical preparation step. This includes dissolving the sample when a solid is analyzed, then separation and purification to isolate the chemical element of interest. This treatment step is crucial to concentrate the sample, eliminate or reduce interferences mainly related to the presence of species with the same mass, and obtain a solution suitable for the element and the measuring device.
An increasing number of laboratories use this technique for the rapid measurement of certain long-lived radionuclides, such as uranium, radium, or thorium. This technique also allows quantifying different isotopes. Measurement by ICP-MS is indeed based on the difference in mass between atoms, and not on their radioactive properties. The sensitivity of the device and the elimination of interferences during measurement allow achieving very low concentrations (even traces), like those present in the environment: up to a few femtograms (10^-15 g) per gram of sample. However, the quantification limit (the smallest measurable mass of the element) depends on the test sample analyzed, the sample matrix, and the radioactive period of the sought radionuclide. Indeed, at equal activity (in Bq), a long-lived radionuclide will have a higher number of atoms, and thus a lower quantification limit.
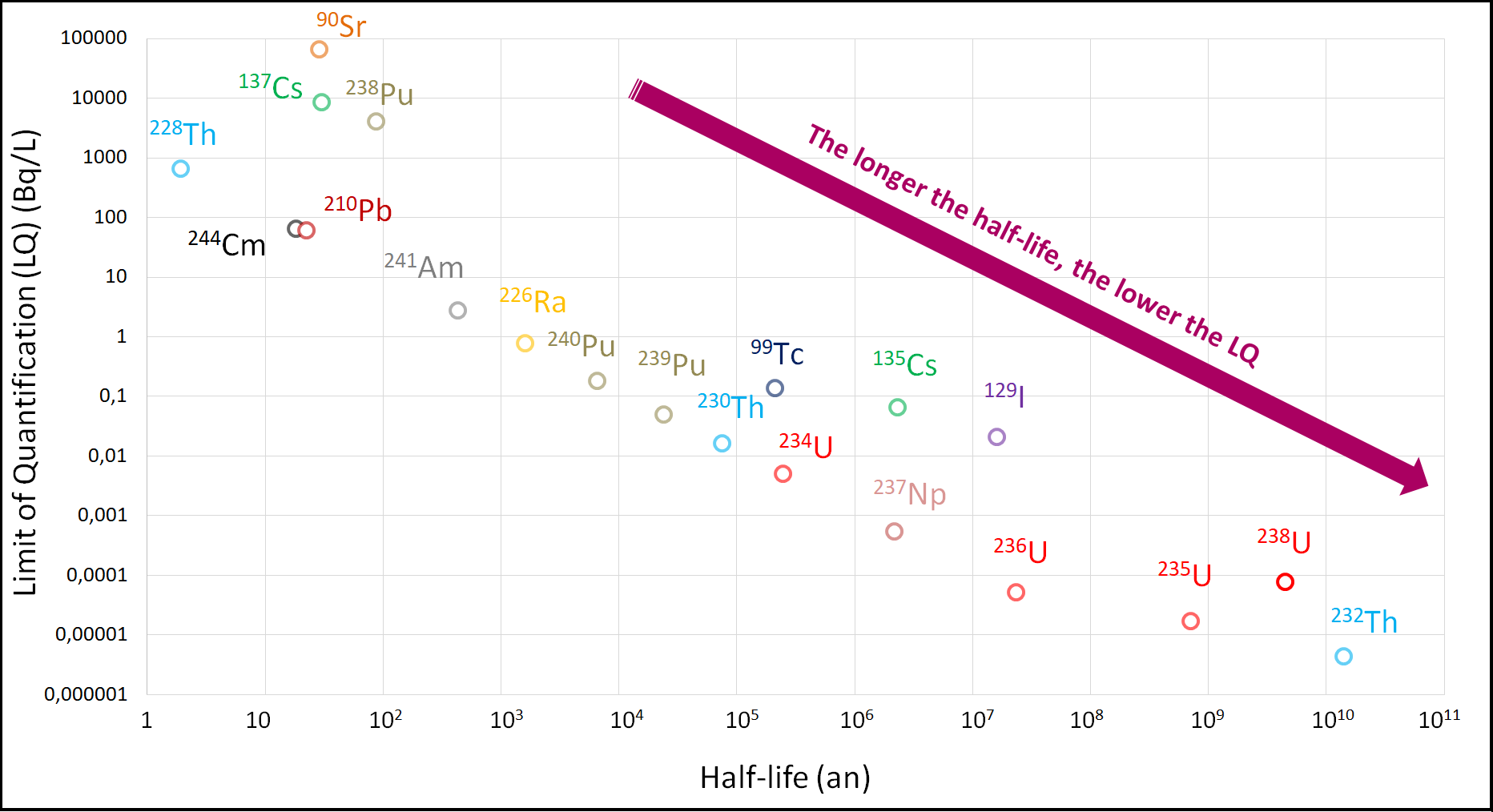
As a result, in the context of analyses carried out for environmental monitoring, the performance of current instruments allows for the low-level quantification of radionuclides with a half-life greater than approximately 1,000 years.
The following figure outlines the operating principle of an ICP-MS:
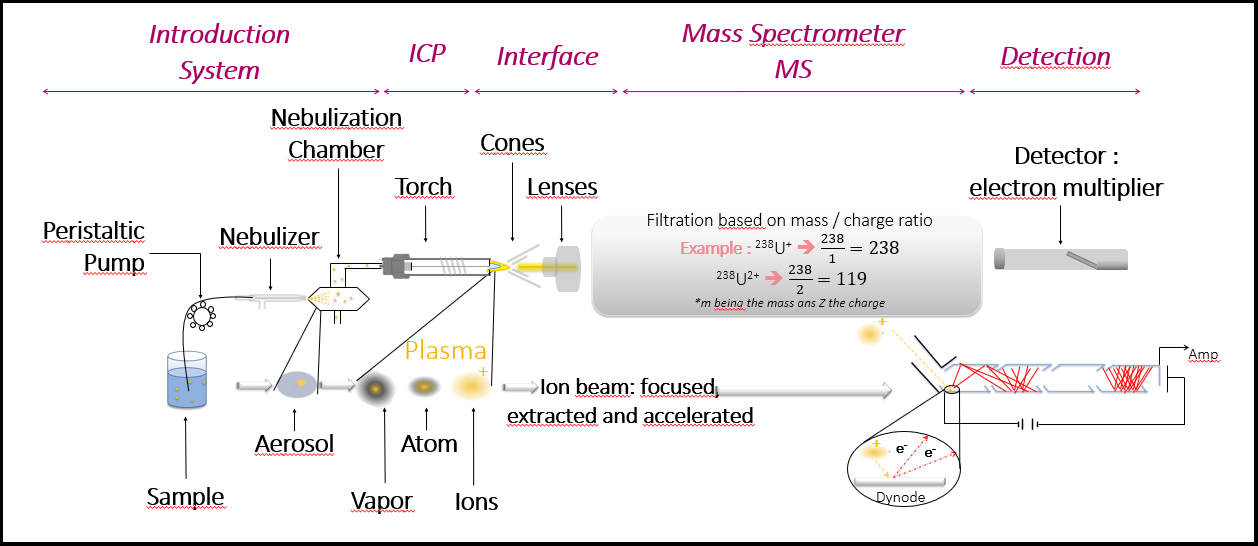
An ICP-MS consists of an introduction system, a spectrometer for ion selection, and a detector. Upon introduction, the liquid sample is sprayed into fine droplets using a carrier gas. Under the effect of an argon plasma, the present species are atomized and the atoms are ionized. The mass spectrometer part then separates the ions according to the mass-to-charge ratio (m/z) to isolate the isotopes of interest. Finally, the detector at the spectrometer's output is an electron multiplier that counts the ions and thus deduces their concentration and activity. The ICP-MS's ability to rapidly scan isotopes allows the analysis of many isotopes in just a few minutes.